Climate Projections in Next Phase of the Coupled Model Intercomparison Project
There is an urgent need for large ensemble climate models with high resolution and fidelity as nations consider local climate mitigation and adaptation efforts for energy efficiency and resource transitioning.
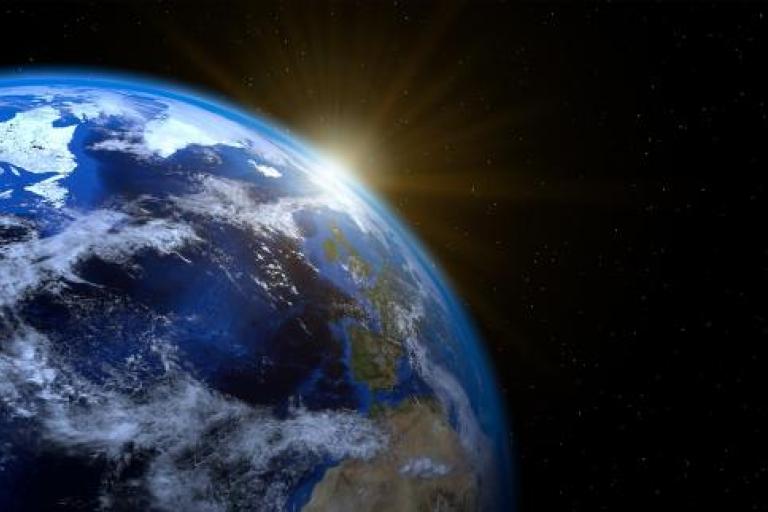
By John Dunne1, Helene Hewitt2, Susann Tegtmeier3, Cath Senior2, Tatiana Ilyina4, Baylor Fox-Kemper5 and Eleanor O’Rourke6
There is an urgent need for large ensemble climate models with high resolution and fidelity as nations consider local climate mitigation and adaptation efforts for energy efficiency and resource transitioning. The Coupled Model Intercomparison Project (CMIP), first launched by World Climate Research Programme (WCRP) in 1995, holds much promise to deliver these in its 7th phase (CMIP7). CMIP6, which is coming to close, made many breakthroughs, especially with Earth System Models (ESMs), but many scientific questions remain unanswered. CMIP7 will endeavour to address these and to find answers for new questions that have arisen, while also focusing on the provision of climate services that will inform local mitigation and adaptation decision-making.
Projections of climate change depend on coupled atmosphere-ocean models to produce simulations of future climate consistent with the underlying physics. Such models are initialized from models or from observations of the current climate state – and driven by scenarios of external forcing such as solar and volcanic forcing and emissions of greenhouse gases, aerosols and land use changes. WCRP spearheaded the effort by establishing CMIP (Meehl, 2023). Structural, scenario and internal variability uncertainties lead to a range of possible outcomes for future climate (Hawkins and Sutton, 2009). CMIP has allowed the uncertainties in future projections from different modelling centres to be characterized and understood. CMIP output has proven essential to the Intergovernmental Panel on Climate Change (IPCC) Assessment Reports of past and possible future changes in climate. Over the years, CMIP projections on regional scales have become increasingly important for policy makers, with the closely related WCRP Coordinated Regional Downscaling Experiment (CORDEX) providing the even greater detail required for decision-making.
Evolving climate and Earth System Models (ESMs)
The main objective of CMIP is to gain a better understanding of past, present and future climate in support of the WCRP science priorities. The earliest coupled atmosphere-ocean models included only “swamp oceans” with the cryosphere and biosphere held fixed (Manabe and Stouffer, 1980). Later generations represented the mass, momentum and energy budgets of the Earth system through a combination of the dynamical equations of motion in atmosphere and ocean, unresolved physical processes, and thermodynamics of the interactions between radiation, clouds, aerosols, cryosphere, ecosystems and human systems.
The increasing societal need for detailed climate change information has driven massive improvements in the modelled representation of the Earth system across resolution – from tens of degrees to now a quarter degree – and comprehensiveness. Current ESMs include representation of physical climate, interactive carbon cycles, chemistry, aerosols, biogeochemistry, ecosystems and human drivers. They also provide an improved granularity via ensembles of models that allow the assessment of structural uncertainty, detection and attribution, predictability, and extremes (Figure 1). As the urgency of climate change has increased over the decades, so has the comprehensiveness of these efforts in parallel with the model improvements (Meehl, 2023).
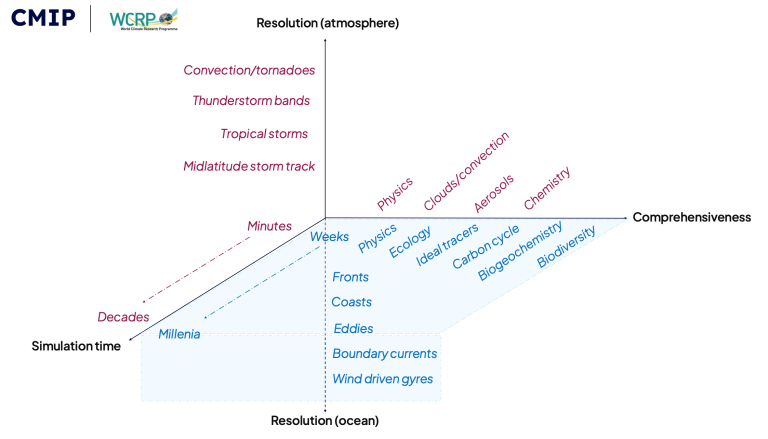
History of climate projections
Climate change projections from climate models were initially based on idealized increases of doubled carbon dioxide (CO2) (Meehl, 2023). Scientists were able to use these experiments to estimate the Equilibrium Climate Sensitivity (Manabe and Stouffer, 1980). They soon added experiments where CO2 was increased by 1% per year to estimate the Transient Climate Response (Mitchell et al., 1990). This was the state of the science in the first and second CMIP phases. These idealized simulations were followed by sets of more realistic historical and projected scenarios as part of the Special Report on Emission Scenarios (1992) used in CMIP3 (Meehl et al., 2004), Representative Concentration Pathways (RCP’s) in CMIP5 (Taylor et al., 2012), and Shared Socio-economic Pathways (SSPs; O’Neill et al., 2016) in CMIP6 (Eyring et al., 2016). In each case, projections included a range of scenarios, from very high emissions to very low, intended to maximally span foreseeable uncertainty. In the IPCC Sixth Assessment Report (AR6; Lee et al., 2021), CMIP6 results provided projections of climate, including changes in key metrics across various realms of the Earth system.
CMIP6 Legacy
To facilitate the efficient and useful application of climate models for multi-model intercomparison, CMIP relies on a distributed and federated infrastructure and community standards to provide model data from coordinated experiments. CMIP6 coordinated 24 individual multi-model science intercomparisons to improve process understanding and assess response to forcing, systematic biases, variability and predictability in line with WCRP Grand Science Challenges (Erying et al., 2016). In this context, over 25 PetaBytes of data were provided and hosted on over 30 data nodes around the globe.
The CMIP6 projections advanced scientific understanding in a number of areas. Highlights include the overall description of climate responses under various scenarios of mitigation (Tebaldi et al., 2020), reduced uncertainty in both effective radiative forcing (Smith et al., 2020) and the climate response with emergent constraints to link inter-model spread and observations (Brient, 2020) with an improved suite of models (e.g. Notz et al., 2020; Roach et al., 2020). CMIP6 provided a robust separation of uncertainty derived from model structure, scenario and internal variability (Lehner et al., 2020), improved quantification of factors that control water availability over land through evaporation and monsoon changes (e.g. Wang et al., 2020), and characterization of risks of climate extremes in temperature and precipitation (Kim et al., 2020). Fully coupled carbon-climate ESMs characterized the Transient Response to Cumulative Emissions (Arora et al., 2020), while interactive chemistry models allowed characterization of short-lived climate forcers in both climate and air pollution (Allen et al., 2020, Turnock et al., 2020). Models representing tropical cyclones were used to estimate future trends (Roberts et al., 2020). One measure of the influence of CMIP6 is the number of citations: there were over 7 500 citations for the CMIP6 overview paper (Eyring et al, 2016) and Scenario Model Intercomparison Project (ScenarioMIP) description (O’Neill et al., 2016) over the last seven years.
Climate model projections delivered through CMIP have provided a wide range of services to the impacts, mitigation and adaptation communities throughout the history of the IPCC. As the utility of models has increased, the physical science and impacts applications have increased exponentially (see article entitled Climate Services Based on Climate Predictions and Projections in this issue). With the current state of climate models capable of representing changing conditions at the continental scale and below, regional downscaling efforts have been eager to apply this information at the national level to support national vulnerability and adaptation planning through efforts such as the Intersectoral Impact Model Intercomparison Project (Warszawski et al., 2014), the Vulnerability, Impacts, Adaptation, and Climate Services Advisory Board (Ruane et al., 2016), the Coordinated Regional Downscaling EXperiment (CORDEX; Giorgi, F., & Gutowski Jr, W. J., 2015) and national assessments. For example, the IPCC AR6 Interactive Atlas is meant to facilitate such efforts, building on CMIP6 and observational data worldwide. These diverse communities have become key partners with CMIP both in the co-development of diagnostic priorities necessary for impact analysis and the iterative identification of model biases limiting these applications. As nations consider mitigation and adaptation efforts such as energy efficiency and source transition, there is increasing need for larger ensembles with high-resolution fidelity to support higher detail in detection and attribution to satisfy more localized and sectoral needs than previously possible.
What is the scientific direction for CMIP7?
Various science questions remain after CMIP6 and new ones have emerged for the next phase (CMIP7) to address. These include questions about the range of climate sensitivity, which became more uncertain among the CMIP6 model ensemble even as the forcing and observational constraints became more certain (Meehl et al., 2020; Figure 2). AR6 also highlighted the deep uncertainty in projections of sea-level rise (Kopp et al., 2023). Better characterization of regional aerosol emissions and variability is critical for realistic simulations of regional temperature, precipitation and air quality responses, which is further complicated by the roles of dust, fire and sea salt. In the longer term, a wide range of potential climate tipping points exist, including large uncertainty in the CO2 and CH4 (methane) responses to soils – particularly microbial mediation in wetlands and permafrost (American Society of Microbiology, 2023) – limiting certainty in remaining carbon budgets and climate stability. The question of climate mitigation effectiveness and unintended consequences have become increasingly pressing and require targeted model experimentation. There is also continued need for better understanding of how extremes and the mapping onto modes of variability, such as the El Niño Southern Oscillation, will change in the future.
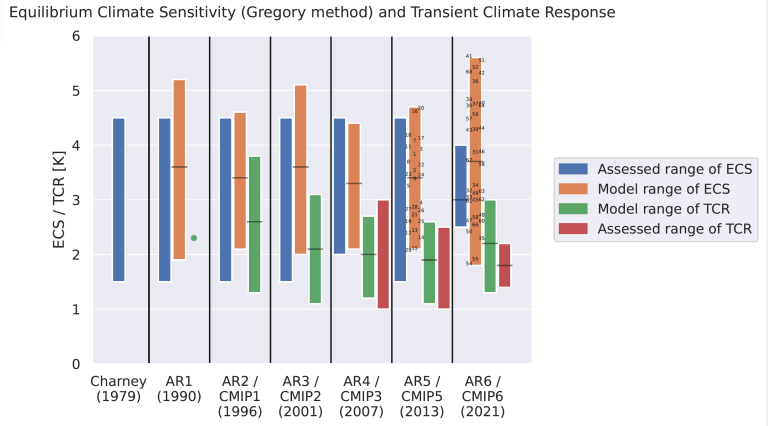
While early climate models required forcing by concentrations of greenhouse gases and relied on Integrated Assessment Models to translate between emissions and concentrations, several modelling centres now have ESMs capable of simulating internally consistent carbon cycles and forcing by CO2 emissions directly (e.g. Figure 3). This capability allows systematic assessment of uncertainties in carbon cycle and carbon-climate feedbacks critical for the estimation of remaining carbon budgets to inform the UNFCCC stocktaking process and to assess the implications of interactions between carbon cycle and climate in the context of “overshoot” and the necessary CO2 removal to reverse climate warming. These ESMs also provide opportunities to explore the implications of alternative pathways for the long-term evolution of the coupled-carbon-climate system.
CMIP7 will have to find the right balance between complexity, resolution and ensemble size (Figure 1). While including complex processes will allow for the full possibility of interactions to occur, increasing model resolution will provide regional details of projections and large ensembles will aid in detecting robust signals in projections. Modelling centres, each with a given high-performance computing resource, prioritize effective use across the dimensions of resolution, complexity, simulation time and the archiving of diagnostics to optimize that resource. Optimizations differ across modelling centres by their mandate and by the “fitness for purpose” of the models themselves – with ESMs having many possible applications to ecosystems, air and water quality, and other impacts beyond physical climate. The centres develop models that are applicable to research questions on climate timescales, meaning models that run at least one, and often tens of, simulation years per day. Even within the focused CMIP objectives on future climate projections, the tension between these dimensions of decision-making lead to a broad diversity in the models as the purpose varies from the impact of extreme storms (high atmospheric horizontal resolution) to stratospheric dynamics and ozone (high atmospheric vertical resolution and interactive chemistry).
CMIP tries to encompass these constraints and address model uncertainty by providing coordination and standardization across climate science and service elements that require coupling between Earth system components. One of the more demanding CMIP data provision requirements comes from dynamical regional downscaling communities such as CORDEX. Data provision challenges will only grow with the new class of global km-scale models coming online (see article Kilometre-Scale Modelling of the Earth system: A new Paradigm for Climate Prediction in this issue). CMIP will need to find a traceable pathway from current capability to embrace new information at smaller spatial and higher temporal scales. Another recent expansion of scope is applications to decadal predictability and prediction through the Decadal Climate Prediction Project. Effectively and efficiently facilitating these and other priority WCRP science activities is a core objective in CMIP.
Future scenario development
The IPCC AR6 Working Group III (Riahi et al., 2022; Figure 3) made considerable advances in a consensus framework for assessment of future scenarios, including defining a baseline of 2021 “implemented policy” (a.k.a. “current policy”) and its relationship to proposed further mitigation pathways. Building on this next generation approach to projections, the ScenarioMIP community met in Reading, United Kingdom (UK), in June to outline the priorities and timelines for generation of an updated suite of future scenarios harmonized to updated historical forcing. Priorities for this suite, outlined in their workshop report, include: continuity with the CMIP6 SSP1–2.6 climate mitigation and SSP3–7.0 climate adaptation/ policy failure scenarios being used by CORDEX, aligning with the AR6 WG III “implemented policy” (Riahi et al., 2022), and expression of scenarios consistent with the Paris 2015 aspiration of keeping warming within 1.5 °C of preindustrial within feasibility constraints. Community presentations and discussions are ongoing along with the establishment of several task teams.
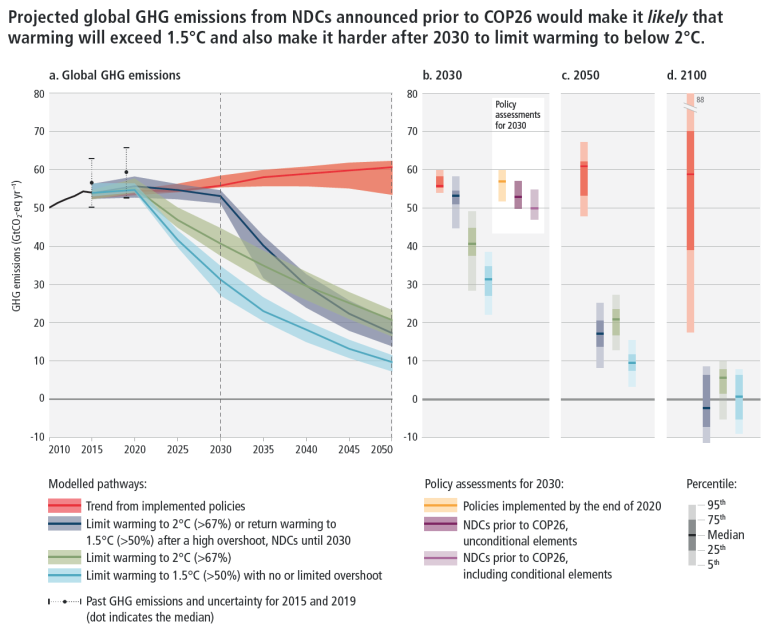
A vision for CMIP into the future
A primary vision for CMIP6 was to have an ongoing model intercomparison project (MIPS; Eyring et al., 2016) to support general scientific advancement beyond the timing and delivery constraints of the IPCC assessment process, which was only partially realized. Moving forward, the CMIP Panel seeks to find ways to realize more fully this combined vision of science and service. Through the newly created International Project Office hosted by European Space Agency, CMIP is actively engaging with interested community members, including modelling centres, infrastructure providers, MIPs, researchers, practitioners, programme managers, IPCC and other stakeholders.
To assist modelling centres with managing resources towards contributing to the IPCC 7th assessment cycle, the CMIP Panel has proposed to identify a harmonized suite of experiments and diagnostics of anticipated priority interest for analysis. CMIP is also moving to a more continuous approach of MIP contributions with independent timelines of experimental design. Ongoing science applications will be assisted by a CMIP task team on forcings that aspires to periodically update historical forcings to facilitate more direct comparison of atmosphere and coupled model simulations with recent historical data.
To enhance and diversify community engagement, CMIP has established a “Fresh Eyes on CMIP” effort to bring insights from early career researchers and practitioners. Finally, only through robust distribution of model data can CMIP continue to provide its research and service role. Efforts are ongoing to secure these resources to leverage state-of-the-art computing and distribution infrastructure to minimize the barriers to efficiently and effectively use CMIP data. In summary, CMIP continues to evolve as an international community effort to support both science and service aspects of coupled modelling.
For further information on CMIP and the development of CMIP7, see https://wcrp-cmip.org
Footnotes
1 National Oceanic and Atmospheric Administration (NOAA) Oceanic and Atmospheric Research (OAR) Division, Geophysical Fluid Dynamics Laboratory (GFLD), United States of America (USA)
2 Met Office, United Kingdom (UK)
3 Institute of Space and Atmospheric Studies, University of Saskatchewan, Canada
4 Centre for Earth System Research and Sustainability (CEN), Hamburg University; Helmholtz-Zentrum Hereon; and Max Planck Institute for Meteorology
5 Department of Earth, Environmental & Planetary Sciences (DEEPS), Brown University
6 World Climate Research Programme (WCRP), Coupled Model Intercomparison Project (CMIP) International Project Office, European Centre for Space Applications and Telecommunications (ECSAT), Harwell Science & Innovation Campus, UK
References:
Allen, R. J., Turnock, S., Nabat, P., Neubauer, D., Lohmann, U., Olivié, D.,... & Collins, W. J. (2020). Climate and air quality impacts due to mitigation of non-methane near-term climate forcers. Atmospheric Chemistry and Physics, 20(16), 9641-9663.
American Society for Microbiology (2023) Microbes in Models: Integrating Microbes into Earth System Models for Understanding Climate Change: Report on an American Academy of Microbiology Virtual Colloquium held on Dec. 6 and 8, 2022. Washington (DC).
Arora, V. K., Katavouta, A., Williams, R. G., Jones, C. D., Brovkin, V., Friedlingstein, P.,... & Ziehn, T. (2020). Carbon–concentration and carbon–climate feedbacks in CMIP6 models and their comparison to CMIP5 models. Biogeosciences, 17(16), 4173-4222.
Brient, F. (2020). Reducing uncertainties in climate projections with emergent constraints: concepts, examples and prospects. Advances in Atmospheric Sciences, 37, 1-15.
Eyring, V., Bony, S., Meehl, G. A., Senior, C. A., Stevens, B., Stouffer, R. J., & Taylor, K. E. (2016). Overview of the Coupled Model Intercomparison Project Phase 6 (CMIP6) experimental design and organization. Geoscientific Model Development, 9(5), 1937-1958.
Giorgi, F., & Gutowski Jr, W. J. (2015). Regional dynamical downscaling and the CORDEX initiative. Annual review of environment and resources, 40, 467-490.
Hawkins, E., & Sutton, R. (2009). The potential to narrow uncertainty in regional climate predictions. Bulletin of the American Meteorological Society, 90(8), 1095-1108.
Kim, Y. H., Min, S. K., Zhang, X., Sillmann, J., & Sandstad, M. (2020). Evaluation of the CMIP6 multi-model ensemble for climate extreme indices. Weather and Climate Extremes, 29, 100269.
Kopp, R.E., Oppenheimer, M., O’Reilly, J.L. et al. (2023) Communicating future sea-level rise uncertainty and ambiguity to assessment users. Nat. Clim. Chang. 13, 648–660. https://doi.org/10.1038/ s41558-023-01691-8
Lee, J.-Y., J. Marotzke, G. Bala, L. Cao, S. Corti, J.P. Dunne, F. Engelbrecht, E. Fischer, J.C. Fyfe, C. Jones, A. Maycock, J. Mutemi, O. Ndiaye, S. Panickal, and T. Zhou, 2021: Future Global Climate: Scenario- Based Projections and Near-Term Information. In Climate Change 2021: The Physical Science Basis. Contribution of Working Group I to the Sixth Assessment Report of the Intergovernmental Panel on Climate Change [Masson-Delmotte, V., P. Zhai, A. Pirani, S.L. Connors, C. Péan, S. Berger, N. Caud, Y. Chen, L. Goldfarb, M.I. Gomis, M. Huang, K. Leitzell, E. Lonnoy, J.B.R. Matthews, T.K. Maycock, T. Waterfield, O. Yelekçi, R. Yu, and B. Zhou (eds.)]. Cambridge University Press, Cambridge, United Kingdom and New York, NY, USA, pp. 553–672, doi:10.1017/9781009157896.006.
Figure SPM.8 Panel A in IPCC, 2021: Summary for Policymakers. In: Climate Change 2021: The Physical Science Basis. Contribution of Working Group I to the Sixth Assessment Report of the Intergovernmental Panel on Climate Change [Masson-Delmotte, V., P. Zhai, A. Pirani, S.L. Connors, C. Péan, S. Berger, N. Caud, Y. Chen, L. Goldfarb, M.I. Gomis, M. Huang, K. Leitzell, E. Lonnoy, J.B.R. Matthews, T.K. Maycock, T. Waterfield, O. Yelekçi, R. Yu, and B. Zhou (eds.)]. Cambridge University Press, Cambridge, UK and New York, NY, USA, pp. 3−32, doi:10.1017/9781009157896.001.]
Lehner, F., Deser, C., Maher, N., Marotzke, J., Fischer, E. M., Brunner, L.,... & Hawkins, E. (2020). Partitioning climate projection uncertainty with multiple large ensembles and CMIP5/6. Earth System Dynamics, 11(2), 491-508.
Wang, B., Jin, C., & Liu, J. (2020). Understanding future change of global monsoons projected by CMIP6 models. Journal of Climate, 33(15), 6471-6489.
Manabe, S. And R. J. Stouffer (1980). Sensitivity of a global climate model to an increase of CO2 concentration in the atmosphere. Journal of Geophysical Research, 85(C10), 5529-5554.
Meehl., G. A., C. Covey, M. Latif, B. McAvaney, J. F. B. Mitchell, and R. Stouffer (2004) Soliciting participation in climate model analyses leading to IPCC Fourth Assessment Report. Eos, Trans. Amer. Geophys. Union, 85, 274
Meehl, G. A., Senior, C. A., Eyring, V., Flato, G., Lamarque, J. F., Stouffer, R. J.,... & Schlund, M. (2020). Context for interpreting equilibrium climate sensitivity and transient climate response from the CMIP6 Earth system models. Science Advances, 6(26), eaba1981.
Meehl, G. A. (2023). The Role of the IPCC in Climate Science. In Oxford Research Encyclopedia of Climate Science.
Mitchell, J. F. B., S. Manabe, V. Meleshko, T. Tokioka, Equilibrium climate change and its implications for the future, in Climate Change: The IPCC Scientific Assessment, J. T. Houghton, G. J. Jenkins, J. J. Ephraums, Eds. (Cambridge Univ. Press, Cambridge, UK, 1990).
Notz, D., & Community, S. I. M. I. P. (2020). Arctic sea ice in CMIP6. Geophysical Research Letters, 47(10), e2019GL086749.
O’Neill, B. C., Tebaldi, C., Van Vuuren, D. P., Eyring, V., Friedlingstein, P., Hurtt, G.,... & Sanderson, B. M. (2016). The scenario model intercomparison project (ScenarioMIP) for CMIP6. Geoscientific Model Development, 9(9), 3461-3482.
Riahi, K., R. Schaeffer, J. Arango, K. Calvin, C. Guivarch, T. Hasegawa, K. Jiang, E. Kriegler, R. Matthews, G.P. Peters, A. Rao, S. Robertson, A.M. Sebbit, J. Steinberger, M. Tavoni, D.P. van Vuuren, 2022: Mitigation pathways compatible with long-term goals. In IPCC, 2022: Climate Change 2022: Mitigation of Climate Change. Contribution of Working Group III to the Sixth Assessment Report of the Intergovernmental Panel on Climate Change [P.R. Shukla, J. Skea, R. Slade, A. Al Khourdajie, R. van Diemen, D. McCollum, M. Pathak, S. Some, P. Vyas, R. Fradera, M. Belkacemi, A. Hasija, G. Lisboa, S. Luz, J. Malley, (eds.)]. Cambridge University Press, Cambridge, UK and New York, NY, USA. doi: 10.1017/9781009157926.005
Roach, L. A., Dörr, J., Holmes, C. R., Massonnet, F., Blockley, E. W., Notz, D.,... & Bitz, C. M. (2020). Antarctic sea ice area in CMIP6. Geophysical Research Letters, 47(9), e2019GL086729.
Ruane, A. C., Teichmann, C., Arnell, N. W., Carter, T. R., Ebi, K. L., Frieler, K.,... & Vincent, K. (2016). The vulnerability, impacts, adaptation and climate services advisory board (VIACS AB v1. 0) contribution to CMIP6. Geoscientific Model Development, 9(9), 3493-3515.
Smith, C. J., Kramer, R. J., Myhre, G., Alterskjær, K., Collins, W., Sima, A.,... & Forster, P. M. (2020). Effective radiative forcing and adjustments in CMIP6 models. Atmospheric Chemistry and Physics, 20(16), 9591-9618.
Tebaldi, C., Debeire, K., Eyring, V., Fischer, E., Fyfe, J., Friedlingstein, P.,... & Ziehn, T. (2020). Climate model projections from the scenario model intercomparison project (ScenarioMIP) of CMIP6. Earth System Dynamics Discussions, 2020, 1-50.
Turnock, S. T., Allen, R. J., Andrews, M., Bauer, S. E., Deushi, M., Emmons, L.,... & Zhang, J. (2020). Historical and future changes in air pollutants from CMIP6 models. Atmospheric Chemistry and Physics, 20(23), 14547-14579.
Warszawski, L., Frieler, K., Huber, V., Piontek, F., Serdeczny, O., & Schewe, J. (2014). The inter-sectoral impact model intercomparison project (ISI–MIP): project framework. Proceedings of the National Academy of Sciences, 111(9), 3228-3232.